Know about the principles instrumentation and applications of Ultraviolet and Visible Spectroscopy
Ultraviolet-visible spectroscopy (UV-Vis) alludes to absorption spectroscopy or reflectance spectroscopy in the ultraviolet-visible spectral region. It uses light in the visible and neighbouring (Near-UV and near-infrared) ranges. The absorption or reflectance in the visible spectrum legitimately influences the apparent shade of the synthetic concoctions included. In this area of the electromagnetic spectrum, atoms experience electronic transitions.
This method is reciprocal to fluorescence spectroscopy, in that fluorescence manages transitions from the excited state to the ground state, while absorption estimates transitions from the ground state to the excited state. This spectroscopy is widely utilised and gave a significant effect on progress in the biochemical and analytical examination. Biomolecules recuperated from column chromatography, enzyme assays, and density gradient centrifugation depends intensely on UV-Vis spectroscopy.
Principle
The majority of the electrons in a particle are in-ground state, and when a compound absorbs UV (200-400 nm) or visible (400-700 nm) radiations, both the bonding and non-bonding outer electron undergo excitation from lower vitality level (ground state) to higher vitality level (excited state). This technique exploits the property of the compound to ingest lights of specific wavelengths which characterises its absorption spectra. The UV-Visible spectrum of a compound gives pieces of information concerning the ground state and the following higher excited state because the good transitions are happening to determine the wavelength of absorbed light — the absorption peaks, identified with deciding the sub-atomic structures.
Instrumentation
A spectrophotometer essentially has the following parts:
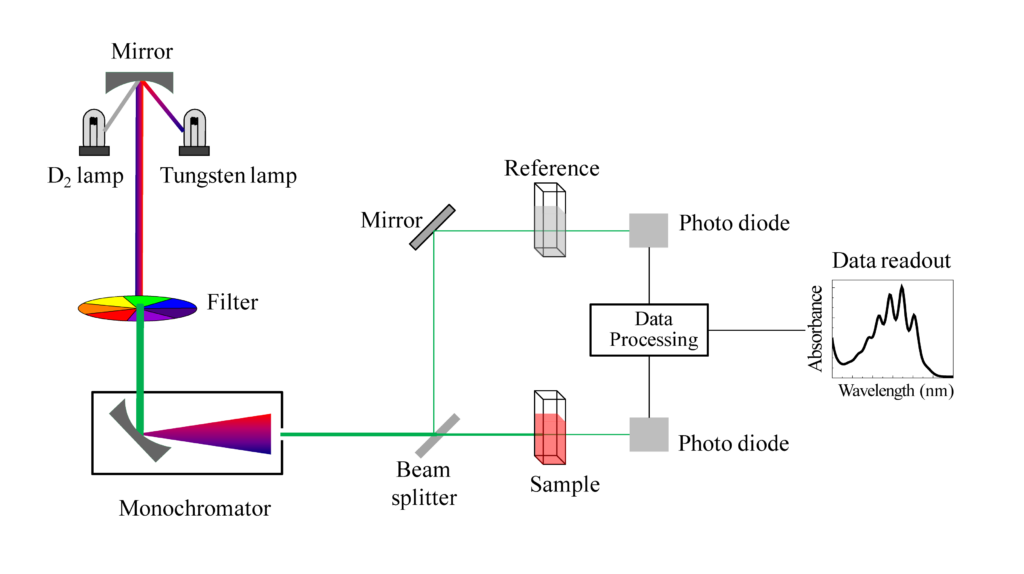
- Light Source
A UV spectrophotometer has two light sources, a tungsten lamp for visible light and a hydrogen or deuterium lamp, and the former gives more extensive and intense light than the latter. This polychromatic light reflected through a plane mirror passes through an entrance slit and a condensing lens and falls on a monochromator. The monochromator disperses the light, and the desired wavelength is focused on the exit slit.
- Monochromators
The monochromators producing radiations of a single wavelength are based either on refraction by a prism or by diffraction by a grating. Prisms are made of glass for the visible region and quartz of silica for the UV region. The resolving power of the grating is directly proportional to the closeness of the lines present in the grating. They are superior to prisms as they yield a linear resolution of the spectrum.
- Cuvettes
The cuvettes are optically transparent made of glass, plastic, silica or quartz. Glass and plastic absorb UV light below 310 nm and hence cannot be used. Silica and quartz do not absorb UV light, and thus they are used for both visible and UV spectrophotometers, and they transit radiation to 110 nm. The standard cuvettes are made of quartz, have an optical path of 1cm and hold a volume of 1 to 3 mL. Microcuvettes – 0.3 mL to 0.5 mL are used for the measurement of expensive chemicals.
- Photomultiplier tubes
A photoelectric device converts light energy into electrical energy which is then amplified, detected and recorded. The photomultiplier tube has a cathode with a photoemissive surface and a wire anode. It also has nine additional cathodes called dynodes. The electrons emitted, from the photoemissive cathode strikes the dynodes which emit several additional electrons. These electrons are accelerated towards dynode two, which again emits electrons, and the amplified electrons flow to the anode generating a much larger photoelectric current than the photocell.
Applications
- UV-Visible spectroscopes are unmistakably progressively refined instruments and give preferable goals and exactness over a colourimeter.
- It is utilised to evaluate the concentration of coloured and colourless solutions, which could retain light.
- Because of its higher susceptibility, it is used to determine even minimal quantities in the matter.
- It, for the most part, does not corrupt or change the substance under examination and henceforth can be recuperated or reused.
- It is utilised to discover the absorption maxima of compounds with a broad scope of wavelengths.
- It additionally empowers to pursue the subtleties of reactions and dynamic enzyme energy.
- It is additionally used to decide the development of bacteria and yeast and decide the number of cells in culture.
- Minimal volumes such as 300 µL, utilised for the estimation of precision samples.
Now that you have read about Ultraviolet and Visible Spectroscopy. Check out our course on Ampersand Academy Read this interesting article about Fourier Transform Infrared Spectroscopy.